如果你也在 怎样代写半导体物理Semiconductor Physics EEE6355这个学科遇到相关的难题,请随时右上角联系我们的24/7代写客服。半导体物理Semiconductor Physics半导体设备可以显示出一系列有用的特性,如电流在一个方向比另一个方向更容易通过,显示出可变电阻,以及对光或热的敏感性。由于半导体材料的电性能可以通过掺杂和应用电场或光来改变,由半导体制成的设备可以用于放大、开关和能量转换。
半导体物理Semiconductor Physics的电导率值介于导体(如金属铜)和绝缘体(如玻璃)之间。它的电阻率随着温度的升高而下降;而金属的表现则相反。它的导电性能可以通过在晶体结构中引入杂质(”掺杂”)的方式进行有用的改变。当同一晶体中存在两个不同的掺杂区域时,就会产生一个半导体结。电荷载体(包括电子、离子和电子空穴)在这些结上的行为是二极管、晶体管和大多数现代电子产品的基础。半导体的一些例子是硅、锗、砷化镓和周期表上所谓 “金属阶梯 “附近的元素。继硅之后,砷化镓是第二种最常见的半导体,被用于激光二极管、太阳能电池、微波频率集成电路等。硅是制造大多数电子电路的一个关键元素。
半导体物理Semiconductor Physics代写,免费提交作业要求, 满意后付款,成绩80\%以下全额退款,安全省心无顾虑。专业硕 博写手团队,所有订单可靠准时,保证 100% 原创。最高质量的半导体物理Semiconductor Physics作业代写,服务覆盖北美、欧洲、澳洲等 国家。 在代写价格方面,考虑到同学们的经济条件,在保障代写质量的前提下,我们为客户提供最合理的价格。 由于作业种类很多,同时其中的大部分作业在字数上都没有具体要求,因此半导体物理Semiconductor Physics作业代写的价格不固定。通常在专家查看完作业要求之后会给出报价。作业难度和截止日期对价格也有很大的影响。
海外留学生论文代写;英美Essay代写佼佼者!
EssayTA™有超过2000+名英美本地论文代写导师, 覆盖所有的专业和学科, 每位论文代写导师超过10,000小时的学术Essay代写经验, 并具有Master或PhD以上学位.
EssayTA™在线essay代写、散文、论文代写,3分钟下单,匹配您专业相关写作导师,为您的留学生涯助力!
我们拥有来自全球顶级写手的帮助,我们秉承:责任、能力、时间,为每个留学生提供优质代写服务
论文代写只需三步, 随时查看和管理您的论文进度, 在线与导师直接沟通论文细节, 在线提出修改要求. EssayTA™支持Paypal, Visa Card, Master Card, 虚拟币USDT, 信用卡, 支付宝, 微信支付等所有付款方式.
想知道您作业确定的价格吗? 免费下单以相关学科的专家能了解具体的要求之后在1-3个小时就提出价格。专家的 报价比上列的价格能便宜好几倍。
想知道您作业确定的价格吗? 免费下单以相关学科的专家能了解具体的要求之后在1-3个小时就提出价格。专家的 报价比上列的价格能便宜好几倍。
我们在物理Physical代写方面已经树立了自己的口碑, 保证靠谱, 高质且原创的物理Physical代写服务。我们的专家在量子力学Quantum mechanics代写方面经验极为丰富,各种量子力学Quantum mechanics相关的作业也就用不着说。

物理代写|半导体物理代写Semiconductor Physics代考|High-Field Properties
In the preceding sections we considered the effect of low electric field on the transport of carriers in semiconductors. In this section we briefly consider some special effects and properties of semiconductors when the electric field is increased to moderate and high levels.
As discussed in Section 1.5.1, at low electric fields the drift velocity in a semiconductor is proportional to the field and the proportionality constant is the mobility that is independent of the electric field. When the fields are sufficiently large, however, nonlinearities in mobility and, in some cases, saturation of drift velocity are observed. At still larger fields, impact ionization occurs. First, we consider the nonlinear mobility.
At thermal equilibrium the carriers both emit and absorb phonons and the net rate of exchange of energy is zero. The energy distribution at thermal equilibrium is Maxwellian. In the presence of an electric field the carriers acquire energy from the field and lose it to phonons by emitting more phonons than are absorbed. At moderately high fields, the most frequent scattering events involve in the emission of acoustic phonons. Thus, the carriers on average acquire more energy than they have at thermal equilibrium. As the field increases, the average energy of the carriers also increases and they acquire an effective temperature $T_e$ that is higher than the lattice temperature $T$. Balancing the rate at which energy is transferred from the field to the carriers by an equal rate of energy loss to the lattice, we obtain from the rate equation, for $\mathrm{Ge}$ and $\mathrm{Si}$ (semiconductors without transferred-electron effect): ${ }^3$
$$
\frac{T_e}{T}=\frac{1}{2}\left[1+\sqrt{1+\frac{3 \pi}{8}\left(\frac{\mu_0 8}{c_s}\right)^2}\right]
$$
and
$$
v_d=\mu_0 \mathscr{E} \sqrt{\frac{T}{T_e}}
$$
where $\mu_0$ is the low-field mobility, and $c_s$ the velocity of sound. For moderate field strength when $\mu_0 \mathscr{E}$ is comparable to $c_s$, the carrier velocity $v_d$ starts to deviate from being linearly dependent on the applied field, by a factor of $\sqrt{T / T_e}$. Finally at sufficiently high fields, carriers start to interact with optical phonons and Eq. 78 is no longer accurate. The drift velocities for Ge and $\mathrm{Si}$ become less and less dependent on the applied field and approach a saturation velocity
$$
v_s=\sqrt{\frac{8 E_p}{3 \pi m_0}} \approx 10^7 \mathrm{~cm} / \mathrm{s}
$$
where $E_p$ is the optical-phonon energy (listed in Appendix G).
物理代写|半导体物理代写Semiconductor Physics代考|Recombination, Generation, and Carrier Lifetimes
Whenever the thermal-equilibrium condition of a semiconductor system is disturbed (i.e., $p n \neq n_i^2$ ), processes exist to restore the system to equilibrium (i.e., $p n=n_i^2$ ). These processes are recombination when $p n>n_i^2$ and thermal generation when $p n<n_i^2$. Figure 25 a illustrates the band-to-band electron-hole recombination. The energy of an electron in transition from the conduction band to the valence band is conserved by emission of a photon (radiative process) or by transfer of the energy to another free electron or hole (Auger process). The former process is the inverse of direct optical absorption, and the latter is the inverse of impact ionization.
Band-to-band transitions are more probable for direct-bandgap semiconductors which are more common among III-V compounds. For this type of transition, the recombination rate is proportional to the product of electron and hole concentrations, given by
$$
R_e=R_{e c} p n .
$$
The term $R_{e c}$, called the recombination coefficient, is related to the thermal generation rate $G_{t h}$ by
$$
R_{e c}=\frac{G_{t h}}{n_i^2} .
$$
$R_{e c}$ is a function of temperature and is also dependent on the band structure of the semiconductor. A direct-bandgap semiconductor, being more efficient in band-toband transitions, has a much larger $R_{e c}\left(\approx 10^{-10} \mathrm{~cm}^3 / \mathrm{s}\right)$ than an indirect-bandgap semiconductor $\left(\approx 10^{-15} \mathrm{~cm}^3 / \mathrm{s}\right)$. In thermal equilibrium, since $p n=n_i^2, R_e=G_{t h}$ and the net transition rate $U\left(=R_e-G_{t h}\right)$ equals zero. Under low-level injection, defined as the case where the excess carriers $\Delta p=\Delta n$ are fewer than the majority carriers, for an $n$-type material $p_n=p_{n o}+\Delta p$ and $n_n \approx N_D$, the net transition rate is given by
$$
\begin{aligned}
U &=R_e-G_{t h}=R_{e c}\left(p n-n_i^2\right) \
& \approx R_{e c} \Delta p N_D \equiv \frac{\Delta p}{\tau_p}
\end{aligned}
$$
where the carrier lifetime for holes
$$
\tau_p=\frac{1}{R_{e c} N_D},
$$
and in $p$-type material,
$$
\tau_n=\frac{1}{R_{e c} N_A} .
$$

半导体物理代写
物理代写|半导体物理代写Semiconductor Physics代考|高场特性
.
在前几节中,我们考虑了低电场对半导体载流子输运的影响。在本节中,我们将简要地讨论当电场增加到中、高水平时半导体的一些特殊效应和性质
如1.5.1节所述,在低电场下,半导体中的漂移速度与电场成正比,比例常数是与电场无关的迁移率。然而,当场足够大时,可观察到流动性的非线性,在某些情况下,漂移速度的饱和。在更大的场中,会发生撞击电离。首先,我们考虑了非线性迁移率
热平衡时,载流子既发射又吸收声子,能量的净交换率为零。热平衡时的能量分布是麦克斯韦式的。在电场存在的情况下,载流子从电场中获得能量,并通过发射比吸收更多的声子而将能量损失给声子。在中等高的场中,最频繁的散射事件涉及声学声子的发射。因此,载流子平均获得比热平衡时更多的能量。随着场的增大,载流子的平均能量也随之增加,载流子获得的有效温度$T_e$高于晶格温度$T$。平衡能量从场转移到载流子的速率与相等的能量损耗到晶格的速率,我们从速率方程中得到,对于$\mathrm{Ge}$和$\mathrm{Si}$(无转移电子效应的半导体):${ }^3$
$$
\frac{T_e}{T}=\frac{1}{2}\left[1+\sqrt{1+\frac{3 \pi}{8}\left(\frac{\mu_0 8}{c_s}\right)^2}\right]
$$
和
$$
v_d=\mu_0 \mathscr{E} \sqrt{\frac{T}{T_e}}
$$
,其中$\mu_0$为低场迁移率,$c_s$为声速。对于中等场强,当$\mu_0 \mathscr{E}$与$c_s$相当时,载流子速度$v_d$开始偏离与应用场强的线性相关的因子$\sqrt{T / T_e}$。最后,在足够高的场下,载流子开始与光学声子相互作用,公式78不再准确。Ge和$\mathrm{Si}$的漂移速度越来越不依赖于应用场,接近饱和速度
$$
v_s=\sqrt{\frac{8 E_p}{3 \pi m_0}} \approx 10^7 \mathrm{~cm} / \mathrm{s}
$$
,其中$E_p$是光声子能量(列于附录G)。
物理代写|半导体物理代写半导体物理学代考|重组、生成和载流子寿命
.
当半导体系统的热平衡条件受到干扰时(即$p n \neq n_i^2$),就会存在恢复系统平衡的过程(即$p n=n_i^2$)。这些过程是$p n>n_i^2$时的重组和$p n<n_i^2$时的产热。图25a显示了带到带电子-空穴复合。一个电子从导带跃迁到价带的能量是通过光子的发射(辐射过程)或通过能量转移到另一个自由电子或空穴(俄歇过程)而守恒的。前者是直接光学吸收的逆过程,后者是碰撞电离的逆过程
直接带隙半导体更有可能发生带间跃迁,这在III-V化合物中更常见。对于这种类型的跃迁,复合速率与电子和空穴浓度的乘积成正比,由
$$
R_e=R_{e c} p n .
$$
给出。术语$R_{e c}$,称为复合系数,与产热速率$G_{t h}$相关,由给出
$$
R_{e c}=\frac{G_{t h}}{n_i^2} .
$$
$R_{e c}$是温度的函数,也依赖于半导体的能带结构。直接带隙半导体在带到带转换中效率更高,其$R_{e c}\left(\approx 10^{-10} \mathrm{~cm}^3 / \mathrm{s}\right)$比间接带隙半导体$\left(\approx 10^{-15} \mathrm{~cm}^3 / \mathrm{s}\right)$大得多。在热平衡中,由于$p n=n_i^2, R_e=G_{t h}$和净跃迁速率$U\left(=R_e-G_{t h}\right)$等于零。在低水平注入下,定义为过量载流子$\Delta p=\Delta n$少于多数载流子的情况下,对于$n$型材料$p_n=p_{n o}+\Delta p$和$n_n \approx N_D$,净跃迁速率由
$$
\begin{aligned}
U &=R_e-G_{t h}=R_{e c}\left(p n-n_i^2\right) \
& \approx R_{e c} \Delta p N_D \equiv \frac{\Delta p}{\tau_p}
\end{aligned}
$$
给出,其中空子的载流子寿命
$$
\tau_p=\frac{1}{R_{e c} N_D},
$$
,而在$p$型材料中,
$$
\tau_n=\frac{1}{R_{e c} N_A} .
$$
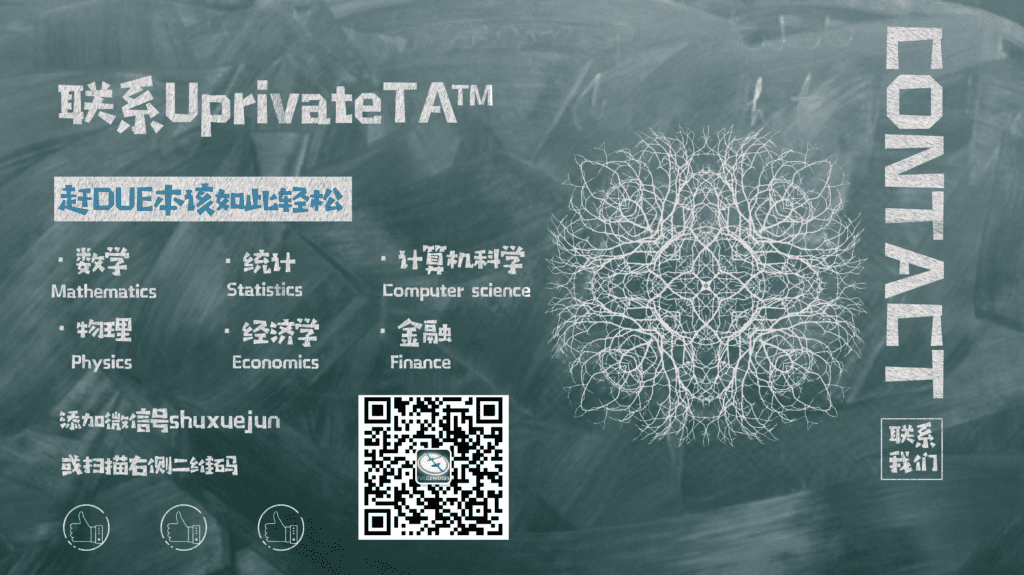
物理代写|半导体物理代写Semiconductor Physics代考 请认准UprivateTA™. UprivateTA™为您的留学生涯保驾护航。
微观经济学代写
微观经济学是主流经济学的一个分支,研究个人和企业在做出有关稀缺资源分配的决策时的行为以及这些个人和企业之间的相互作用。my-assignmentexpert™ 为您的留学生涯保驾护航 在数学Mathematics作业代写方面已经树立了自己的口碑, 保证靠谱, 高质且原创的数学Mathematics代写服务。我们的专家在图论代写Graph Theory代写方面经验极为丰富,各种图论代写Graph Theory相关的作业也就用不着 说。
线性代数代写
线性代数是数学的一个分支,涉及线性方程,如:线性图,如:以及它们在向量空间和通过矩阵的表示。线性代数是几乎所有数学领域的核心。
博弈论代写
现代博弈论始于约翰-冯-诺伊曼(John von Neumann)提出的两人零和博弈中的混合策略均衡的观点及其证明。冯-诺依曼的原始证明使用了关于连续映射到紧凑凸集的布劳威尔定点定理,这成为博弈论和数学经济学的标准方法。在他的论文之后,1944年,他与奥斯卡-莫根斯特恩(Oskar Morgenstern)共同撰写了《游戏和经济行为理论》一书,该书考虑了几个参与者的合作游戏。这本书的第二版提供了预期效用的公理理论,使数理统计学家和经济学家能够处理不确定性下的决策。
微积分代写
微积分,最初被称为无穷小微积分或 “无穷小的微积分”,是对连续变化的数学研究,就像几何学是对形状的研究,而代数是对算术运算的概括研究一样。
它有两个主要分支,微分和积分;微分涉及瞬时变化率和曲线的斜率,而积分涉及数量的累积,以及曲线下或曲线之间的面积。这两个分支通过微积分的基本定理相互联系,它们利用了无限序列和无限级数收敛到一个明确定义的极限的基本概念 。
计量经济学代写
什么是计量经济学?
计量经济学是统计学和数学模型的定量应用,使用数据来发展理论或测试经济学中的现有假设,并根据历史数据预测未来趋势。它对现实世界的数据进行统计试验,然后将结果与被测试的理论进行比较和对比。
根据你是对测试现有理论感兴趣,还是对利用现有数据在这些观察的基础上提出新的假设感兴趣,计量经济学可以细分为两大类:理论和应用。那些经常从事这种实践的人通常被称为计量经济学家。
MATLAB代写
MATLAB 是一种用于技术计算的高性能语言。它将计算、可视化和编程集成在一个易于使用的环境中,其中问题和解决方案以熟悉的数学符号表示。典型用途包括:数学和计算算法开发建模、仿真和原型制作数据分析、探索和可视化科学和工程图形应用程序开发,包括图形用户界面构建MATLAB 是一个交互式系统,其基本数据元素是一个不需要维度的数组。这使您可以解决许多技术计算问题,尤其是那些具有矩阵和向量公式的问题,而只需用 C 或 Fortran 等标量非交互式语言编写程序所需的时间的一小部分。MATLAB 名称代表矩阵实验室。MATLAB 最初的编写目的是提供对由 LINPACK 和 EISPACK 项目开发的矩阵软件的轻松访问,这两个项目共同代表了矩阵计算软件的最新技术。MATLAB 经过多年的发展,得到了许多用户的投入。在大学环境中,它是数学、工程和科学入门和高级课程的标准教学工具。在工业领域,MATLAB 是高效研究、开发和分析的首选工具。MATLAB 具有一系列称为工具箱的特定于应用程序的解决方案。对于大多数 MATLAB 用户来说非常重要,工具箱允许您学习和应用专业技术。工具箱是 MATLAB 函数(M 文件)的综合集合,可扩展 MATLAB 环境以解决特定类别的问题。可用工具箱的领域包括信号处理、控制系统、神经网络、模糊逻辑、小波、仿真等。