如果你也在 怎样代写太阳系Solar System FENG2021这个学科遇到相关的难题,请随时右上角联系我们的24/7代写客服。太阳系Solar System是由太阳和围绕太阳运行的物体组成的引力约束系统。它在46亿年前由一个巨大的星际分子云的引力坍缩形成。该系统的绝大部分(99.86%)质量都在太阳中,其余大部分质量包含在木星中。内系统的四颗行星–水星、金星、地球和火星–是陆生行星,主要由岩石和金属组成。
太阳系Solar System系统的四颗巨行星比陆生行星大得多,质量也大得多。两个最大的行星,木星和土星,是气态巨行星,主要由氢和氦组成;接下来的两个行星,天王星和海王星,是冰态巨行星,主要由与氢和氦相比熔点较高的挥发性物质组成,如水、氨和甲烷。所有八颗行星都有近乎圆形的轨道,位于地球轨道的平面附近,称为黄道。
太阳系Solar System代写,免费提交作业要求, 满意后付款,成绩80\%以下全额退款,安全省心无顾虑。专业硕 博写手团队,所有订单可靠准时,保证 100% 原创。最高质量的太阳系Solar System作业代写,服务覆盖北美、欧洲、澳洲等 国家。 在代写价格方面,考虑到同学们的经济条件,在保障代写质量的前提下,我们为客户提供最合理的价格。 由于作业种类很多,同时其中的大部分作业在字数上都没有具体要求,因此太阳系Solar System作业代写的价格不固定。通常在专家查看完作业要求之后会给出报价。作业难度和截止日期对价格也有很大的影响。
海外留学生论文代写;英美Essay代写佼佼者!
EssayTA™有超过2000+名英美本地论文代写导师, 覆盖所有的专业和学科, 每位论文代写导师超过10,000小时的学术Essay代写经验, 并具有Master或PhD以上学位.
EssayTA™在线essay代写、散文、论文代写,3分钟下单,匹配您专业相关写作导师,为您的留学生涯助力!
我们拥有来自全球顶级写手的帮助,我们秉承:责任、能力、时间,为每个留学生提供优质代写服务
论文代写只需三步, 随时查看和管理您的论文进度, 在线与导师直接沟通论文细节, 在线提出修改要求. EssayTA™支持Paypal, Visa Card, Master Card, 虚拟币USDT, 信用卡, 支付宝, 微信支付等所有付款方式.
想知道您作业确定的价格吗? 免费下单以相关学科的专家能了解具体的要求之后在1-3个小时就提出价格。专家的 报价比上列的价格能便宜好几倍。
想知道您作业确定的价格吗? 免费下单以相关学科的专家能了解具体的要求之后在1-3个小时就提出价格。专家的 报价比上列的价格能便宜好几倍。
我们在物理Physical代写方面已经树立了自己的口碑, 保证靠谱, 高质且原创的物理Physical代写服务。我们的专家在量子力学Quantum mechanics代写方面经验极为丰富,各种量子力学Quantum mechanics相关的作业也就用不着说。

物理代写|太阳系代写Solar System代考|Early Stages of Planetary Growth
Dust grains are a relatively minor constituent of protoplanetary disks, but they represent the starting point for the formation of rocky planets like Earth, and possibly also gas-rich planets like Jupiter. These grains are small, typically $1 \mu \mathrm{m}$ in diameter or less. In a microgravity environment, electrostatic forces dominate interactions between such grains. Charge transfer during grain collisions can lead to the formation of grain dipoles that align with one another forming aggregates up to several centimeters in size. Freshly deposited frost surfaces make grains stickier and increase the ability of grain aggregates to hold together during subsequent collisions.
Laboratory experiments show that low-velocity collisions between grains tend to result in sticking, while faster collisions often cause grains to rebound. Irregularly shaped micron-sized grains often stick to one another at collision speeds of up to tens of meters per second. Fluffy aggregates may stick more readily than compact solids as some of the energy of impact goes into compaction. However, the primary components of chondritic meteorites are compact chondrules, so further compaction cannot have played a big role in the formation of their parent bodies. In general, sticking forces scale with the surface area of an object, while collisional energy scales with mass and hence volume. As a result, growth becomes more difficult, and breakup becomes more likely, as aggregates become larger. It is possible that early growth in the solar nebula took place mainly as the result of large objects sweeping up smaller ones. This idea is supported by recent experiments that found that small dust aggregates tend to embed themselves in larger ones if they collide at speeds above about $10 \mathrm{~m} / \mathrm{s}$.
Dust grains, grain aggregates, and chondrules would have been closely coupled to the motion of gas in the solar nebula. The smallest particles were mainly affected by Brownian motion-collisions with individual gas molecules, which caused the particles to move with respect to one another, leading to collisions. Particles also settled slowly toward the disk’s midplane due to the vertical component of the Sun’s gravitational field. Settling was opposed by gas drag so that each particle fell at its terminal velocity:
$$
v_{\mathrm{z}}=-\left(\frac{\rho}{\rho_{\text {gas }}}\right)\left(\frac{v_{\mathrm{kep}}}{c_{\mathrm{s}}}\right)\left(\frac{r z}{a^2}\right) v_{\mathrm{kep}}
$$
where $r$ and $\rho$ are the radius and density of the particle, $\rho_{\text {gas }}$ is the gas density, $a$ is the orbital distance from the Sun, $z$ is the height above the disk midplane, and $c_{\mathrm{s}}$ is the sound speed in the gas. Here $v_{\text {kep }}$ is the speed of a solid body moving on a circular orbit, called the Keplerian velocity:
$$
v_{\mathrm{kep}}=\sqrt{\frac{G M_{\text {sun }}}{a}}
$$
where $M_{\text {sum }}$ is the mass of the Sun. Large particles fell faster than small ones, sweeping up material as they went, increasing their vertical speed further. Calculations show that micron-sized particles would grow and reach the midplane in about $10^3-10^4$ orbital periods if these were the only processes operating.
物理代写|太阳系代写Solar System代考|Formation of Terrestrial Planets
The growth of bodies beyond $1 \mathrm{~km}$ in size is reasonably well understood. Gravitational interactions and collisions between pairs of planetesimals dominate the evolution from this point onward. A key factor in determining the rate of growth is gravitational focusing. The probability that two planetesimals will collide during a close approach depends on their cross-sectional area multiplied by a gravitational focusing factor $F_{\mathrm{g}}$ :
$$
F_{\mathrm{g}}=1+\frac{v_{\mathrm{esc}}^2}{v_{\mathrm{rel}}^2}
$$
where $v_{\text {rel }}$ is the planetesimals’ relative velocity, and $v_{\text {esc }}$ is the escape velocity from a planetesimal, given by
$$
v_{\text {esc }}=\sqrt{\frac{2 G M}{r}}
$$
where $M$ and $r$ are the planetesimal’s mass and radius, respectively. When planetesimals pass each other slowly, there is time for their mutual gravitational attraction to focus their trajectories toward each other, so $F_{\mathrm{g}}$ is large, and the chance of a collision is high. Fast moving bodies typically do not collide unless they are traveling directly toward each other because $F_{\mathrm{g}} \cong 1$ in this case. The relative velocities of planetesimals depend on their orbits about the Sun. Objects with similar orbits are the most likely to collide with each other. In particular, planetesimals moving on nearly circular, coplanar orbits have high collision probabilities while ones with highly inclined, eccentric (elliptical) orbits do not.
Most close encounters between planetesimals did not lead to a collision, but bodies often passed close enough for their mutual gravitational tug to change their orbits. Statistical studies show that after many such close encounters, high-mass bodies tend to acquire circular, coplanar orbits, while low-mass bodies are perturbed onto eccentric, inclined orbits. This is called dynamical friction and is analogous to the equipartition of kinetic energy between molecules in a gas. Dynamical friction means that, on average, the largest bodies in a particular region experience the strongest gravitational focusing; therefore, they grow the fastest (Fig. 17). This state of affairs is called runaway growth for obvious reasons. Most planetesimals remained much larger.

太阳系代写
物理代写|太阳系代写太阳系代考|行星生长的早期阶段
尘粒是原行星盘中相对次要的组成部分,但它们代表着像地球这样的岩质行星的形成起点,也可能是像木星这样富含气体的行星的形成起点。这些颗粒很小,通常直径为$1 \mu \mathrm{m}$或更小。在微重力环境中,静电力主导着这些颗粒之间的相互作用。粒子碰撞过程中的电荷转移会导致形成相互对齐的粒子偶极子,形成高达几厘米大小的聚集体。新沉积的霜表面使颗粒更粘,并增加了在随后的碰撞中颗粒聚集在一起的能力
实验室实验表明,颗粒之间的低速碰撞往往会导致粘着,而更快的碰撞往往会导致颗粒反弹。不规则形状的微米大小的颗粒经常以高达每秒几十米的碰撞速度相互粘在一起。松软的集料比致密的固体更容易粘在一起,因为撞击的一些能量进入了压实过程。然而,球粒陨石的主要成分是致密的球粒,因此进一步的压实作用不可能在它们母体的形成中发挥很大作用。一般来说,粘着力与物体的表面积成正比,而碰撞能量则与质量和体积成正比。其结果是,随着总量的增加,增长变得更加困难,解体的可能性也更大。太阳星云的早期生长可能主要是大物体扫向小物体的结果。这一观点得到了最近实验的支持,实验发现,如果小的尘埃聚集体以大约$10 \mathrm{~m} / \mathrm{s}$以上的速度碰撞,它们往往会嵌入较大的尘埃聚集体中
尘埃颗粒、颗粒聚集物和球粒可能与太阳星云中的气体运动密切相关。最小的粒子主要受到与单个气体分子的布朗运动碰撞的影响,这导致粒子相对于其他粒子运动,从而导致碰撞。由于太阳引力场的垂直分量,粒子也缓慢地向圆盘的背板沉降。沉降受到气体阻力的阻碍,因此每个粒子以其终端速度下落:
$$
v_{\mathrm{z}}=-\left(\frac{\rho}{\rho_{\text {gas }}}\right)\left(\frac{v_{\mathrm{kep}}}{c_{\mathrm{s}}}\right)\left(\frac{r z}{a^2}\right) v_{\mathrm{kep}}
$$
其中$r$和$\rho$是粒子的半径和密度,$\rho_{\text {gas }}$是气体密度,$a$是到太阳的轨道距离,$z$是高于圆盘背板的高度,$c_{\mathrm{s}}$是气体中的声速。这里$v_{\text {kep }}$是一个固体在圆形轨道上运动的速度,称为开普勒速度:
$$
v_{\mathrm{kep}}=\sqrt{\frac{G M_{\text {sun }}}{a}}
$$
其中$M_{\text {sum }}$是太阳的质量。大粒子的下落速度比小粒子快,在运动过程中会把物体卷起来,从而进一步提高垂直速度。计算表明,如果这些是唯一运行的过程,微米大小的粒子将在大约$10^3-10^4$个轨道周期内生长并到达背板
物理代写|太阳系代写太阳系代考|类地行星的形成
超过$1 \mathrm{~km}$大小的物体的增长已经被相当好地理解了。引力相互作用和成对星子之间的碰撞从这一点开始主导着演化。决定生长速率的一个关键因素是引力聚焦。两个星子在近距离接近时发生碰撞的概率取决于它们的截面积乘以引力聚焦因子$F_{\mathrm{g}}$:
$$
F_{\mathrm{g}}=1+\frac{v_{\mathrm{esc}}^2}{v_{\mathrm{rel}}^2}
$$
,其中$v_{\text {rel }}$是星子的相对速度,$v_{\text {esc }}$是来自星子的逃逸速度,由
$$
v_{\text {esc }}=\sqrt{\frac{2 G M}{r}}
$$
给出,其中$M$和$r$分别是星子的质量和半径。当星子彼此缓慢通过时,它们之间的相互引力有时间使它们的轨迹聚焦到彼此身上,所以$F_{\mathrm{g}}$很大,碰撞的几率很高。快速运动的物体通常不会碰撞,除非它们直接向对方运动,因为在这种情况下$F_{\mathrm{g}} \cong 1$。星子的相对速度取决于它们围绕太阳的轨道。轨道相似的物体最容易相互碰撞。特别是,在近圆形共面轨道上运动的星子有很高的碰撞概率,而高度倾斜、偏心(椭圆)轨道的星子则没有。大多数星子之间的近距离接触不会导致碰撞,但物体经常经过得足够近,以至于它们的相互引力牵引改变了它们的轨道。统计研究表明,在多次这样的近距离接触后,高质量天体倾向于获得圆形的共面轨道,而低质量天体则被扰动到偏心的倾斜轨道上。这被称为动力摩擦,类似于气体中分子之间动能的均分。动力摩擦意味着,平均而言,在特定区域最大的物体经历最强的引力聚焦;因此,它们生长最快(图17)。这种情况被称为“失控增长”,原因很明显。大多数星子仍然要大得多
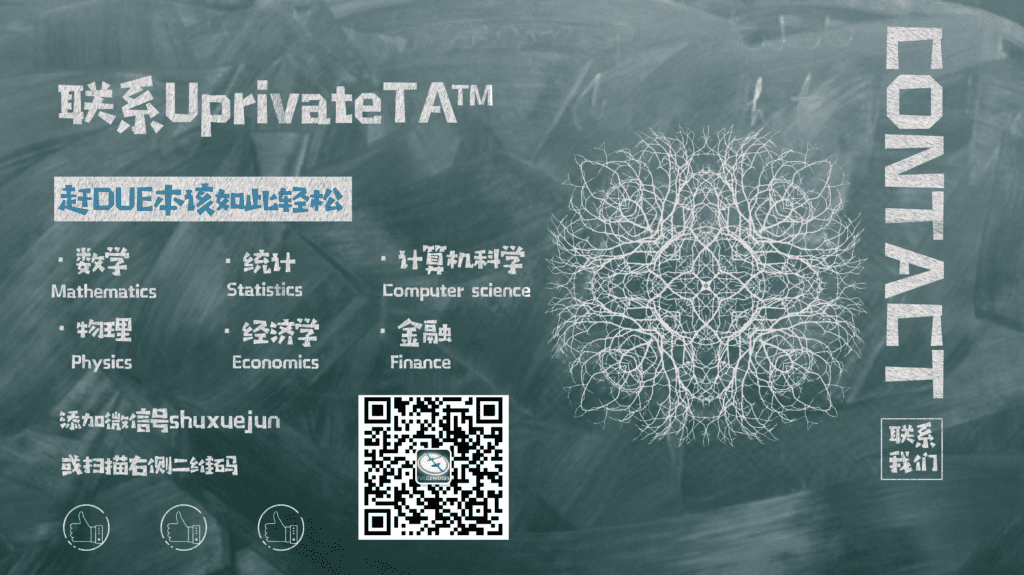
物理代写|太阳系代写Solar System代考 请认准UprivateTA™. UprivateTA™为您的留学生涯保驾护航。
微观经济学代写
微观经济学是主流经济学的一个分支,研究个人和企业在做出有关稀缺资源分配的决策时的行为以及这些个人和企业之间的相互作用。my-assignmentexpert™ 为您的留学生涯保驾护航 在数学Mathematics作业代写方面已经树立了自己的口碑, 保证靠谱, 高质且原创的数学Mathematics代写服务。我们的专家在图论代写Graph Theory代写方面经验极为丰富,各种图论代写Graph Theory相关的作业也就用不着 说。
线性代数代写
线性代数是数学的一个分支,涉及线性方程,如:线性图,如:以及它们在向量空间和通过矩阵的表示。线性代数是几乎所有数学领域的核心。
博弈论代写
现代博弈论始于约翰-冯-诺伊曼(John von Neumann)提出的两人零和博弈中的混合策略均衡的观点及其证明。冯-诺依曼的原始证明使用了关于连续映射到紧凑凸集的布劳威尔定点定理,这成为博弈论和数学经济学的标准方法。在他的论文之后,1944年,他与奥斯卡-莫根斯特恩(Oskar Morgenstern)共同撰写了《游戏和经济行为理论》一书,该书考虑了几个参与者的合作游戏。这本书的第二版提供了预期效用的公理理论,使数理统计学家和经济学家能够处理不确定性下的决策。
微积分代写
微积分,最初被称为无穷小微积分或 “无穷小的微积分”,是对连续变化的数学研究,就像几何学是对形状的研究,而代数是对算术运算的概括研究一样。
它有两个主要分支,微分和积分;微分涉及瞬时变化率和曲线的斜率,而积分涉及数量的累积,以及曲线下或曲线之间的面积。这两个分支通过微积分的基本定理相互联系,它们利用了无限序列和无限级数收敛到一个明确定义的极限的基本概念 。
计量经济学代写
什么是计量经济学?
计量经济学是统计学和数学模型的定量应用,使用数据来发展理论或测试经济学中的现有假设,并根据历史数据预测未来趋势。它对现实世界的数据进行统计试验,然后将结果与被测试的理论进行比较和对比。
根据你是对测试现有理论感兴趣,还是对利用现有数据在这些观察的基础上提出新的假设感兴趣,计量经济学可以细分为两大类:理论和应用。那些经常从事这种实践的人通常被称为计量经济学家。
MATLAB代写
MATLAB 是一种用于技术计算的高性能语言。它将计算、可视化和编程集成在一个易于使用的环境中,其中问题和解决方案以熟悉的数学符号表示。典型用途包括:数学和计算算法开发建模、仿真和原型制作数据分析、探索和可视化科学和工程图形应用程序开发,包括图形用户界面构建MATLAB 是一个交互式系统,其基本数据元素是一个不需要维度的数组。这使您可以解决许多技术计算问题,尤其是那些具有矩阵和向量公式的问题,而只需用 C 或 Fortran 等标量非交互式语言编写程序所需的时间的一小部分。MATLAB 名称代表矩阵实验室。MATLAB 最初的编写目的是提供对由 LINPACK 和 EISPACK 项目开发的矩阵软件的轻松访问,这两个项目共同代表了矩阵计算软件的最新技术。MATLAB 经过多年的发展,得到了许多用户的投入。在大学环境中,它是数学、工程和科学入门和高级课程的标准教学工具。在工业领域,MATLAB 是高效研究、开发和分析的首选工具。MATLAB 具有一系列称为工具箱的特定于应用程序的解决方案。对于大多数 MATLAB 用户来说非常重要,工具箱允许您学习和应用专业技术。工具箱是 MATLAB 函数(M 文件)的综合集合,可扩展 MATLAB 环境以解决特定类别的问题。可用工具箱的领域包括信号处理、控制系统、神经网络、模糊逻辑、小波、仿真等。